Key Priorities for Water Electrolyzer Material & Component Innovation
Feb 28, 2024
Chingis Idrissov
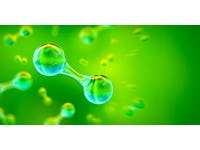
The production of green hydrogen continues to be the focus of many governmental and corporate decarbonization strategies. The electrolyzer is a key technology that enables green hydrogen production by splitting water molecules into hydrogen and oxygen using renewable electricity. Many focus on the need for large-scale green hydrogen plants as well as electrolyzer manufacturing facilities. However, material and component innovation is often given less priority in the broader market. This article will shed light on some critical material and component priorities for the key electrolyzer technologies using insights from the IDTechEx report, "Materials for Green Hydrogen Production 2024-2034: Technologies, Players, Forecasts".
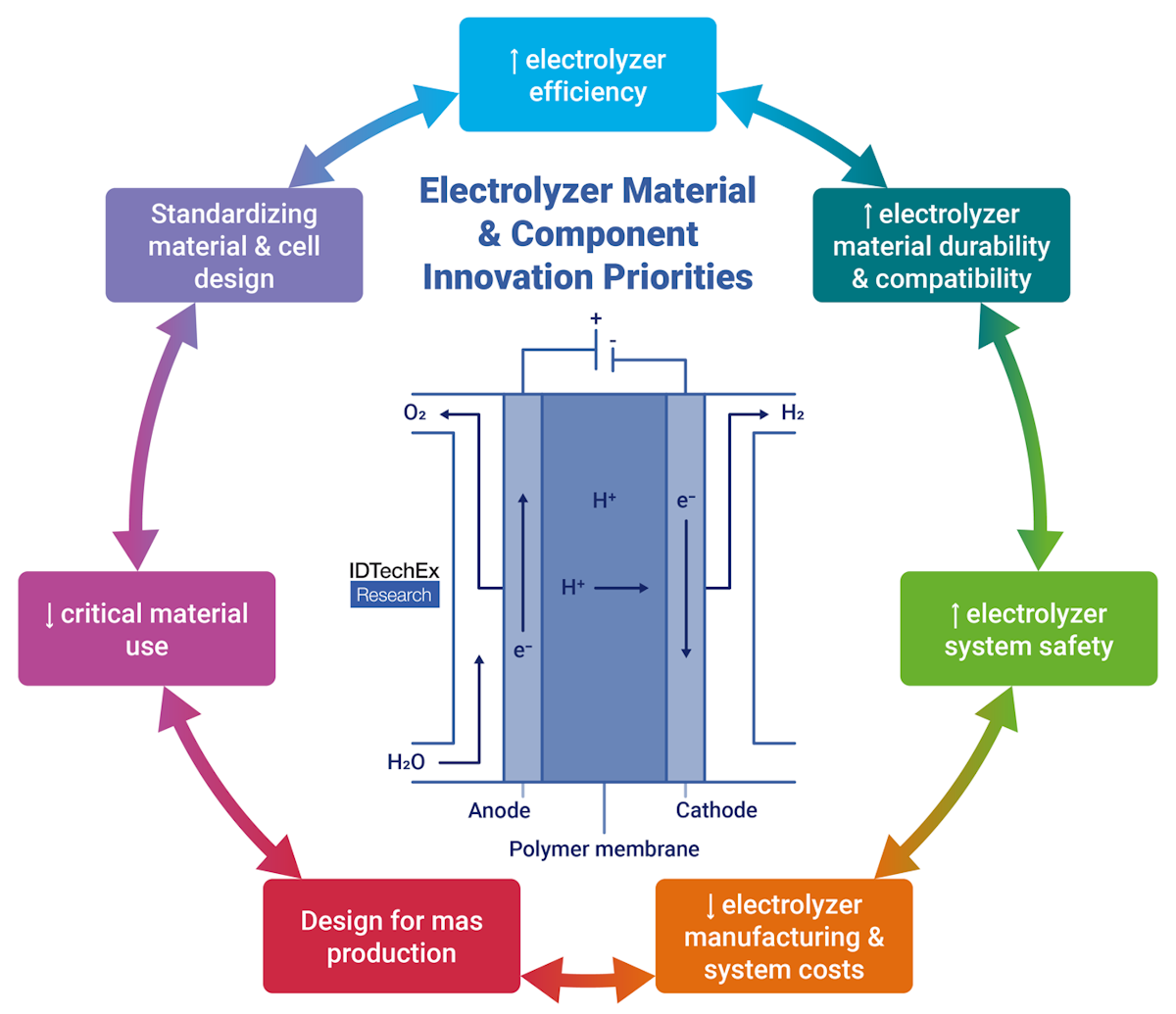
Electrolyzer material and component innovation priorities. Source: IDTechEx
Alkaline water electrolyzer (AWE): new electrode designs for improved efficiency
The alkaline water electrolyzer (AWE) is a mature and highly commercialized technology, providing the lowest system capital cost and predictable long-term performance. The AWE cell stack uses a liquid alkaline electrolyte (potassium hydroxide, KOH) coupled with a porous diaphragm and relies on widely available materials, such as nickel and stainless steel.
One of the key challenges with AWE is its lower efficiency compared to its main competitor technology - the proton exchange membrane electrolyzer (PEMEL). As a result, electrolyzer technology and material developers have focused on producing high-performance catalytic coatings for electrodes to improve the conversion efficiency of water into hydrogen and oxygen. High-performance coatings exist commercially, but many still rely on the use of critical platinum group metals (PGMs), such as ruthenium, in mixed oxide coatings.
Research into electrode coatings has been focused on discovering and applying new catalytic materials. Promising strategies include coupling the incumbent nickel with other transition and non-transition metal elements, for instance in NiFeCoP. Moreover, the use of porous electrodes, such as nickel meshes, can couple particularly well with such coatings, producing highly nano-structured catalysts that provide high surface areas for reactions.
Pressing these electrodes against the porous diaphragm can further improve efficiency due to the reduced distance that chemical species must travel between reaction sites. This is known as the zero-gap cell configuration and is already widely employed in commercial AWE stacks. De Nora is one of the zero-gap cell components and advanced electrode coatings and is working with established AWE suppliers such as McPhy Energy and thyssenkrupp nucera. IDTechEx expects that combining these material and component design strategies may further improve the AWE's efficiency, but more commercial efforts are needed across the board.
Proton exchange membrane electrolyzer (PEMEL): reducing the need for expensive & critical materials
Proton exchange membrane electrolyzers (PEMEL) are gaining significant traction for their high efficiency, compact design, and adaptability with fluctuating renewable energy sources. While there is a movement towards standardizing materials in PEMEL stacks, innovation is far from stagnant, particularly in anode catalyst development.
Platinum (Pt) and iridium (Ir) catalyze the electrochemical hydrogen and oxygen evolution reactions occurring in the electrolyzer, respectively. The state-of-the-art materials used are platinum supported on carbon black (Pt/C) for the cathode and iridium black (Ir) or iridium oxide (IrOx) for the anode. The use of iridium at the anode is particularly problematic, as current loadings of 1 - 2.5 g/kW and high demand for PEMEL systems may put a significant strain on global iridium supply.
Catalyst suppliers, such as Heraeus Precious Metals, are addressing the iridium issue by coupling iridium with ruthenium in a mixed oxide catalyst (IrRuOx). Given the pressing issue of reducing iridium loading, this material may see heightened commercial uptake in the near future. Another strategy being explored both in academic and corporate R&D groups is the use of supported iridium catalysts, similar to the Pt/C approach. Furthermore, much of the research is focused on eliminating iridium in favor of other PGMs, such as ruthenium, by coupling it with transition metals in mixed oxides. Iridium and PGM-free catalysts are still generally at an earlier stage of development. IDTechEx predicts that more research efforts would be needed to demonstrate their competitiveness and pave the path for widespread commercial adoption.
Other components in the PEM electrolyzer, such as the gas diffusion layers (GDLs) and bipolar plates also use precious metal coatings as well as titanium. Both can become more problematic in the future, so many companies are exploring new manufacturing and coating methods to reduce requirements for these materials. For more information, please refer to IDTechEx's previous research article on PEM electrolyzer materials.
Anion exchange membrane electrolyzer (AEMEL): improving membranes & avoiding critical materials
The anion exchange membrane electrolyzer (AEMEL) is a relatively young but growing technology aiming to combine the best of both alkaline and PEM technologies. AEMEL seeks to blend the material abundance of the AWE with the high-efficiency characteristic of the PEMEL. This technology is experiencing rapid growth and innovation, exemplified by companies like Enapter, which are pioneering commercial megawatt-scale systems.
IDTechEx has noted that many research groups studying AEMEL technology still use platinum and iridium-based catalysts at the electrodes. A key focus of the industry and new commercial players should be to fully eliminate the use of PGMs and opt for high-performance nano-structured catalysts used in the AWE space. Furthermore, this technology could benefit from the application of gas diffusion electrodes (GDEs), which combine the gas diffusion layer with the electrode into a single component.
Another issue is the wide variation in anion exchange membrane (AEM) materials and, hence, their varied performance and durability. AEMs can benefit from some of their strategies seen in the proton exchange membrane (PEM) space, such as the cross-linking of polymer chains and the use of supporting layers (e.g., PTFE) onto which the AEM can be coated. The variation in materials is likely to persist, but AEMEL developers can take inspiration from the current developments seen in AWE and PEMEL technologies, applying them to their own cell and stack designs.
Solid oxide electrolyzer (SOEC): optimization of cell structure for high performance & low degradation
The solid oxide electrolyzer (SOEC) is also a relatively new technology in the electrolysis landscape, with a smaller market presence compared to AWE and PEMEL. The SOEC operates at much higher temperatures (>600°C) than the previously mentioned low-temperature technologies (50-90°C) and, therefore, requires vastly different materials and design approaches.
SOEC technology has massively benefitted from material developments in the solid oxide fuel cell (SOFC) technology as most stacks are designed to operate reversibly, that is either as an electrolyzer (SOEC) or fuel cell (SOFC). While certain ceramic components have already been established in the technology, the development of new materials and electrode-electrolyte assemblies in SOECs is a promising area for innovation.
Innovation priorities include the use of electrolytes that enable lower operating temperatures that can provide long-term stability and cost benefits. Among them is gadolinia-doped ceria (GDC), which is already used in some commercial stacks and exemplified by companies like Ceres Power. Another key focus is the use of metal-supported cells (MSCs) due to several inherent advantages over electrode- or electrolyte-supported cells, which are more common in commercial stacks. These include reduced material costs as well as the ability to better withstand thermal cycling and resist mechanical stresses. Furthermore, many efforts are going into improving the thermal and chemical compatibility of the cell materials with interconnects, contact layers, and sealants.
Future SOEC stacks may see the adoption of more advanced materials. Overall, there is a wide range of materials used in SOEC stacks, which not only highlights the diversity in the technology but also shows the potential for material innovation in these high-temperature electrolyzers.
Market outlook & strategic insights
Electrolyzer technology and material developers face numerous challenges, many of which are interrelated. For example, reducing the thickness of the proton or anion exchange membrane can enhance efficiency but may reduce the membrane's durability. Enhancing durability through advanced materials and manufacturing techniques, on the other hand, increases manufacturing costs.
Consequently, there is no single optimal approach to materials and components innovation. However, this process of optimization opens significant business opportunities for the development of more sophisticated electrolyzer materials.
The electrolyzer component market is poised for significant expansion, with IDTechEx's projections estimating its market value to reach an impressive US$31.7 billion by 2034. This growth is predominantly driven by the rapidly evolving green hydrogen industry, where electrolyzers play a crucial role. In the "Materials for Green Hydrogen Production 2024-2034: Technologies, Players, Forecasts" report by IDTechEx, a thorough analysis is presented on the current and future materials and components used in the four electrolyzer technologies discussed in this article. The report offers a breakdown of stack manufacturing costs by component for AWE, PEMEL, and SOEC stacks. Comprehensive lists of electrolyzer stack, component, and material suppliers are available, with case studies of key commercial innovations in the industry. Moreover, it provides granular 10-year market forecasts, quantifying the demand for materials and components in tonnes, square meters (m2), and US$ million annually.
To find out more about this IDTechEx report, including downloadable sample pages, please visit www.IDTechEx.com/GreenHydrogen.
IDTechEx guides your strategic business decisions through its Research, Subscription and Consultancy products, helping you profit from emerging technologies. For more information, contact research@IDTechEx.com or visit www.IDTechEx.com.